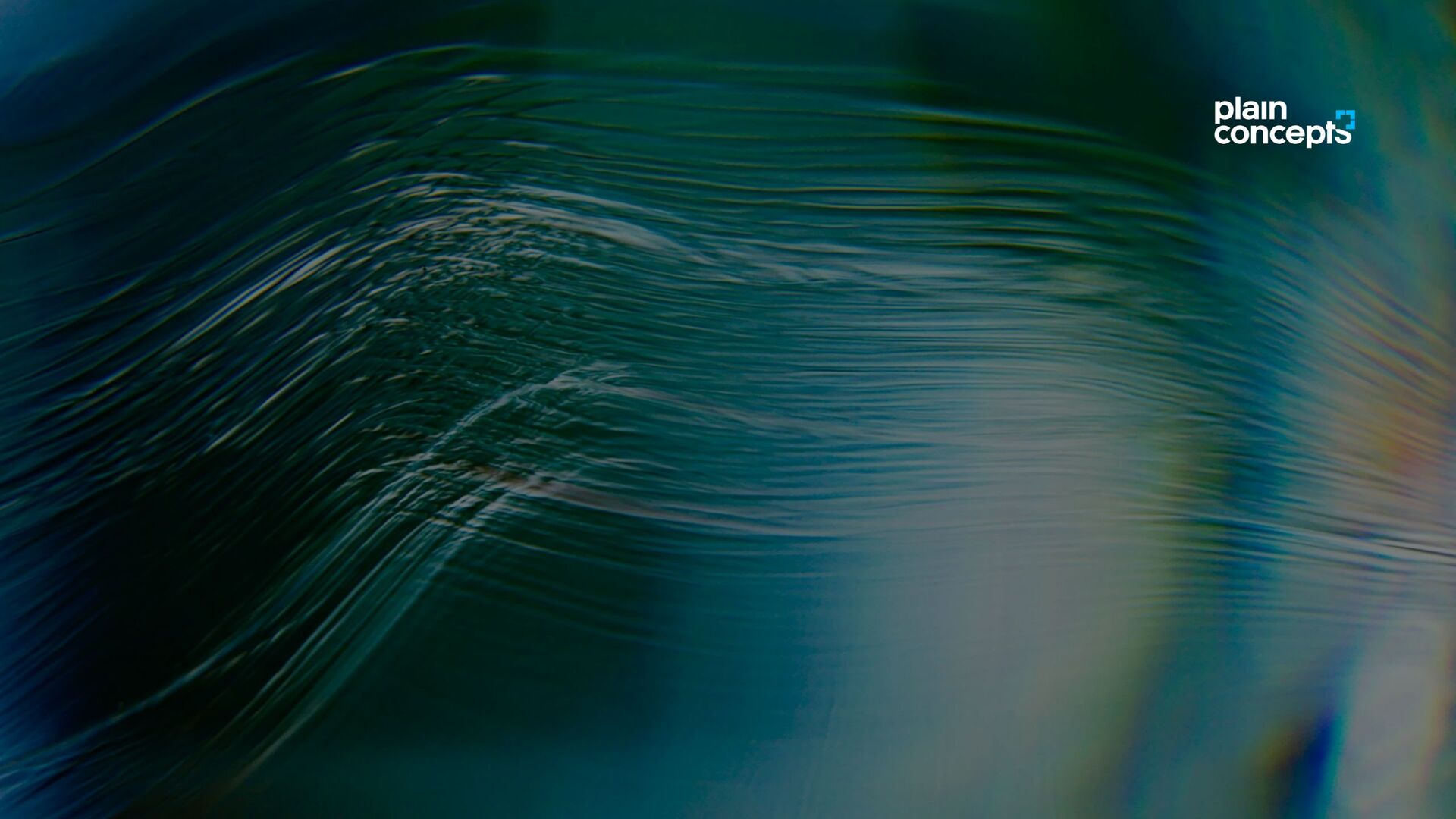
Quantum Computing: Potential and Challenges ahead
According to McKinsey, the quantum computing market could grow to around $80 billion by 2035 or 2040. We are currently at a point where many qubit technologies are competing to become the basis for the first universal, faultless quantum computer, but there are still many challenges to be faced.
We look at its future prospects, its potential, and the impact it will have on individuals and businesses.
Getting into Quantum Computing
A few months ago we shared with you other articles on whether companies should prepare for quantum computing and the fundamentals around Quantum Computing.
In short, quantum computing harnesses the power of quantum mechanics to help us overcome the limitations that today’s computing world has been encountering. However, because the quantum state of a particle is a natural phenomenon, it means that it can be reproduced in many different ways.
It has the potential to transform many industries, as such computers solve problems exponentially faster and with less power consumption than traditional computers.
As a result, it will allow companies to better optimize their investment strategies, encryption, or discover new products. And some of its most widespread applications will be in cryptography, data analysis, predictions, pattern search, drug research, etc.
A key turning point in the history of quantum computing has been reached with the concept of “quantum supremacy”, which refers to the point at which a quantum computer outperforms the most powerful classical supercomputers. This achievement shows how quantum computers have the ability to tackle complicated problems ten times faster than classical computers.
In fact, in 2019, Google reached a turning point in this area by achieving quantum supremacy, indicating that the use of quantum computers for useful purposes is feasible.
Qubit technology evaluation
No approach has yet emerged to evaluate and compare qubit technologies that can produce a perfect quantum computer. However, we did find 6 key considerations and challenges for evaluating them:
- Fidelity to scale: this is closely related to the defining constraint of quantum computing, which is to increase the number of qubits and the computational power for complex algorithms while maintaining high levels of quality.
- Computational speed: individual qubits can retain their quantum state (coherence) only for a limited time, so to compensate, gating operations should occur fast enough to make complex computations possible before qubits lose coherence.
- Multi-qubit networks: the more qubits that can be linked together, the more easily quantum computing algorithms can be implemented and the more powerful the resulting computer will be.
- Control over individual qubits at scale: this control is fundamental, but as the number of qubits in a quantum computing system increases, control over individual qubits becomes increasingly complex.
- Cooling and environmental control: the required scale of cooling equipment in terms of size and power is beyond the feasibility of currently available equipment.
- Manufacturing: some qubit designs use existing production technology, while others require new manufacturing techniques. Therefore, such production will require automated manufacturing and testing of components at scale.
Core qubit technologies
Because the industry and the technology are still at a relatively early stage of development, McKinsey has chosen the five main qubit technologies:
- Photonic networks: In these networks, each qubit is encoded in a single photon. An initial cluster state is prepared and then the gate operations are executed through a series of photon measurements. They have great potential for massive quantum entanglement, speed, and ability to operate at room temperature. In contrast, the major source of error is the loss of photons during calculations and their source.
- Superconducting circuits: work by encoding each qubit in the energy levels of Cooper pairs on opposite sides of a Josephon junction. Their benefits are coherence, processing speed, and ease of fabrication. In addition, they can continue to be used as the technology matures and more qubits are added. However, scaling, calibration, control electronics, and cooling are challenging.
- spin qubits: In spin qubit systems, each qubit is encoded in the electron spin of a semiconductor quantum dot. Two-qubit gates are established between entangled electrons on a silicon chip, and the qubits are controlled by microwave electronics. Their small size and simplicity of fabrication are big advantages, but they face many of the challenges of superconductors, such as scale fidelity and control electronics.
- Neutral atoms: In this technology, a qubit is encoded in two hyperfine ground-state levels of an atom. Its advantages range from scaling to coherence and cooling, but its challenges center on scaling to a million qubits, control electronics, and error rates, which are not yet clearly possible.
- Trapped ions: here each qubit is encoded in two energy level states of an ion. Two-qubit gates exploit the coupling between the electron and phonon, between the excited electron state of an ion, and the vibrational modes of the ionic chain. These trapped ions have the benefit of the lowest error rates among the technologies we examined for small two-qubit gate systems, as well as lower cooling requirements compared to other atom-based technologies. Fidelity at scale is also less of a challenge, as qubits can be physically moved and manipulated. However, the increase of qubits in these systems is the most important obstacle.
Challenges of Quantum Computing
Despite remarkable advances, quantum computing still faces many technological hurdles that limit its applications, scalability, and reliability for the time being.
Due to their fragility, qubit interconnection, decoherence, and external noise, quantum systems are prone to errors. Therefore, there is a need to improve fault-tolerant quantum structures, qubit coherence times, and error correction methods.
On the other hand, the creation of quantum hardware (cryogenic cooling systems, control circuits, and qubit fabrication) remains a technical challenge that requires interdisciplinary cooperation and creativity.
Decoherence
Compared to classical computers, quantum computers are extremely susceptible to noise, as the quantum state of qubits is very fragile and any perturbation, such as a slight vibration or a change in temperature, can affect them uncontrollably, causing the stored information to be lost.
Decoherence poses a challenge in quantum computing, since, in order to avoid errors, calculations must be completed before decoherence occurs. While increasing the coherence time of a qubit is an important area of research, a popular approach to address it is the development of error-correcting codes.
While error-correcting codes are not a new concept, they become much more challenging in the quantum environment than in the classical one and can manifest themselves in phase shifts, bit shifts, or a combination of both. Furthermore, qubits cannot be replicated in the same way as classical bits, requiring much more complex correction codes.
In this process, quantum information stored in a logical qubit is protected from errors by encoding it in several physical qubits. There are now many widely used error-correction codes, each of which represents a different way of encoding quantum information, such as the Shor code, the Steane code, or the Surface code.
Scalability
Scalability refers to the ability to increase the number of qubits in a quantum system to enable more complex problems to be solved.
It is a very complex process due to the difficulty of connecting a large number of qubits to create larger systems. One possible solution is the use of error correction techniques that involve creating redundancy in the system to allow for error detection and correction.
However, to be successful, these techniques require the use of additional qubits and complex algorithms, which can further complicate the scaling process.
Physical implementation
There are different methods for building quantum computers, such as superconducting circuits, trapped ions, and topological qubits. Each method has its own difficulties, and it is not yet clear which will be the most feasible for large-scale quantum computing.
Cost and Accessibility
Currently, quantum computers are expensive and require very specialized environments to operate. Therefore, one of the big challenges for this technology is to make it accessible for widespread use.
Workforce and experience
The field of quantum computing requires a deep understanding of quantum mechanics, computer science, and engineering, profiles in which there is already a shortage of individual talent.
Finding professionals with expertise in all three is difficult, which may slow down the development and adoption of quantum computing technologies.
Quantum Computing and AI
As we have discussed previously, quantum computing has the potential to revolutionize many fields, and the marriage of quantum computing and artificial intelligence is particularly promising.
Although we are still in the early stages of understanding the implementation of AI with quantum technology, numerous potential benefits and innovations can arise from this synergy, including significant advances in AI capabilities.
According to Capitol Technology University, some of the areas in which quantum computing could enhance AI include:
- Parallelism and quantum acceleration: Quantum computers exploit the principles of superposition and entanglement, allowing them to perform multiple computations simultaneously. This parallelism could lead to a significant acceleration of AI algorithms, especially for tasks that involve processing large datasets or solving complex optimization problems.
- Optimization and ML: Quantum computing is very useful for solving optimization problems prevalent in Machine Learning. Quantum algorithms (such as QAOA) could be used to improve the optimization tasks involved in training ML models, making the processes faster and more efficient.
- Improved data processing: Quantum computers excel at handling and processing large amounts of data due to their inherent parallelism. This capability could benefit AI applications that require extensive data processing, such as PLN, image recognition, and recommender systems.
- Enhanced encryption and security: Quantum technology also poses a potential threat to current encryption methods, but can also be used to enhance security in AI applications. One example is using quantum-resistant cryptographic techniques to safeguard sensitive data, ensuring the privacy and security of AI systems.
- Exploring quantum neural networks: Quantum neural networks are an area of research that aims to combine the principles of quantum computing with neural network architectures. These could offer new ways of modeling and representing complex data, which could lead to more robust and expressive AI models.
- Simulation of quantum systems: Quantum computers are adept at simulating quantum systems, which could be beneficial for AI applications related to quantum chemistry, materials science, and drug discovery. This capability could lead to advances in understanding complex molecular interactions and the design of new materials.
- Energy efficiency: this type of computing has the potential to solve problems with far fewer steps than classical computers, which could lead to more energy-efficient AI algorithms, especially for tasks that currently require extensive computational resources.
As for the possible challenges and the future of quantum-powered AI, they are the same as computing itself, such as scalability, error correction or software and programming.
Future of Quantum Computing
Despite the challenges facing quantum computing, its future looks promising. Technology giants such as IBM, Google, and Microsoft, along with other research companies, are investing large sums of money in the field.
It will become a fundamental tool for scientific research, making it easier to solve problems that were previously impossible.
Researchers are making continuous progress in increasing the coherence times of qubits, reducing error rates, and developing new quantum algorithms. As a result, governments are also beginning to see the strategic importance of quantum computing, resulting in increased funding and collaborative efforts.
As the technology matures, it will be crucial to keep abreast of developments in quantum computing. It is very important to identify these use cases early on, as well as to clarify the challenges involved in managing expectations. They can be proven to work on a small scale and, as quantum computers grow in qubits, the same algorithms can be run on larger amounts of data. The growth of quantum capacity is exponential, so every computer that gets one more qubit will double its computational capacity.
At Plain Concepts we can help you prepare for this new wave of technology that will change the way we do business as we know it.